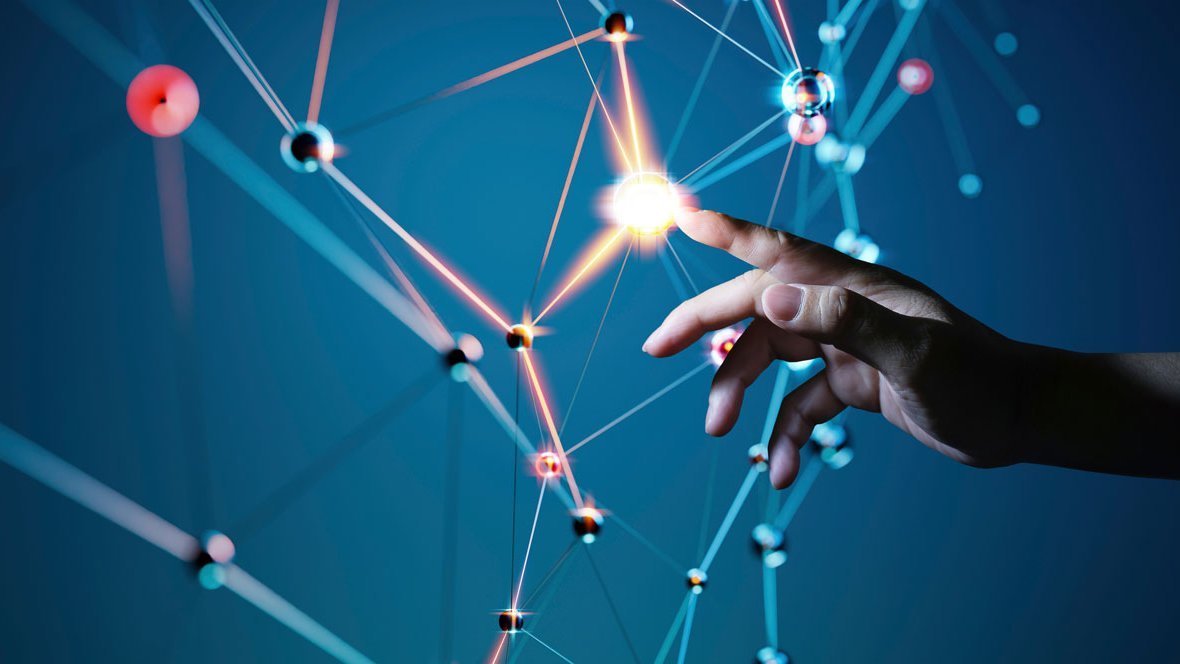
触觉全息术或空中触觉技术有望让虚拟现实成为现实,但一项新研究揭示了一个令人意外的物理障碍,有待克服。加州大学圣巴巴拉分校的一个研究团队发现了一种新现象,它是新兴全息触觉显示器的基础,这一发现可能会带来更引人入胜的虚拟现实体验。该团队的研究成果还发表在《科学进展》杂志上,获得了高度认可。
全息触觉显示器使用超声发射器相控阵,在空中聚焦超声波,用户无需借助物理设备或接口,就能徒手触摸、感受并操控空中的三维虚拟物体。虽然这些显示器在增强现实、虚拟现实和远程呈现等众多应用领域前景广阔,但目前它们提供的触感较为分散、微弱,感觉就像 “微风” 或 “一口气”。
加州大学圣巴巴拉分校工程学院副教授扬・维塞尔表示:“我们的新研究解释了为什么这类全息图的触感比预期的要分散、模糊得多。” 他的研究重点是交互技术,尤其侧重于触觉、机器人学和电子学领域。
由扬・维塞尔和博士生研究员格雷戈里・里尔登牵头的这项研究,运用激光测振、模拟和感知实验,全面研究了触觉全息过程中皮肤中出现的超声激发波。他们发现,全息显示器会在皮肤中激发广泛的振动模式,即所谓的剪切激波。
维塞尔解释说,在触觉全息术中,当超声波在空中聚焦和扫描时会产生激波,进而引起皮肤振动。这些振动会相互干扰,在某些位置增强振动强度,这种现象被称为相长干涉。激波的形成会产生一种拖尾尾流模式,延伸到预期焦点之外,降低了触感的空间精度和清晰度。研究人员打了个比方,若聚焦的声束是水面上快速行驶的船,那么激波模式就是船后的尾流。目前的全息触觉显示器激发的激波模式在皮肤中过于分散,导致触感非常模糊。
研究人员使用Polytec公司的 PSV扫描式激光测振仪,在用户感受全息触觉反馈时,对手部表面进行振动成像。测量结果揭示了皮肤中超声诱发的剪切激波模式的显著表现。
维塞尔说:“我们的研究表明,全息触觉显示器作为一种很有前景的虚拟现实和远程呈现新技术,需要声学领域的新知识和设计创新。通过了解皮肤中超声产生的剪切激波的基本物理原理,我们希望改进全息触觉显示器的设计,让用户获得更逼真、更沉浸的体验。”
这类触觉显示器能让我们用无数虚拟物体、互动动画角色或可抓取工具来丰富物理环境,这些虚拟元素不仅能被看到,还能用手触摸和感知。
在通过数值模拟预测出剪切激波后,维塞尔团队利用扫描式激光测振仪将聚焦超声在皮肤上引发的真实波型可视化,以此验证模拟结果。随后,他们开展感知实验,将波型与用户感知关联起来,评估其对用户体验的影响。
图 1 展示了实验装置。扫描式激光测振仪捕捉到的表面振荡,是由图中绿色部分所示的超声换能器相控阵发射聚焦超声,刺激具有类似人体皮肤力学特性的组织模型产生的(图 1A)。最后,在人体手部 300 多个测点进行了振动测量(图 1B)。
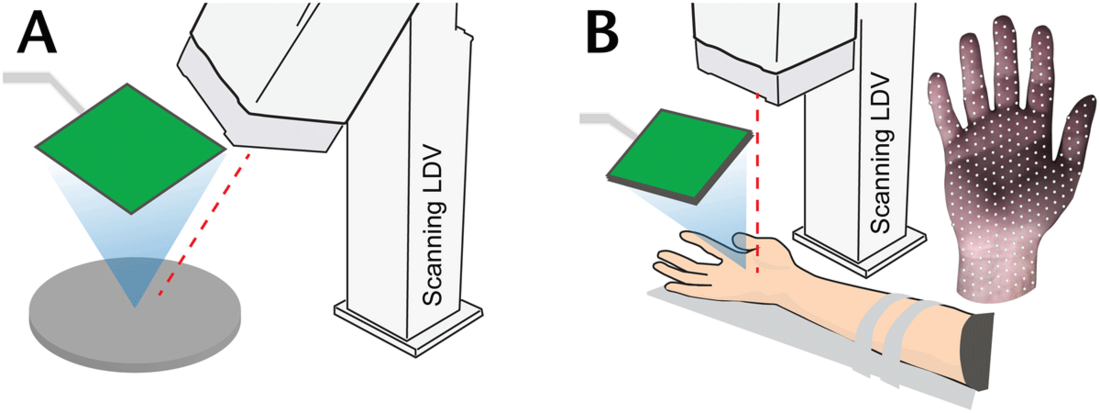
当超声源以跨音速到超音速的速度在表面扫描时,会激发出大振幅剪切激波,其尾流角度与速度相关(图 2 右图),这与波动力学以及数值模拟结果一致,。这些剪切激波模式滞后于焦点位置,在几毫秒内迅速形成。其尾流区域的表面积比有效声学焦点面积大几个数量级。因此,有效聚焦分辨率主要由激波形成决定,而非超声聚焦。波能在长度 10 cm或更长的尾流中跟随焦点位置,还会在垂直于扫描路径的方向上延伸数cm。较低的扫描速度(v = 2 m/s,图 2 左图)产生的波型因粘性阻尼部分集中在焦点附近。然而,这些波的振幅较小、频率较低,皮肤只能微弱地感知到。
图 2 中的插图展示了激发波型的频率成分。随着马赫数增加,激发的高频范围变宽。
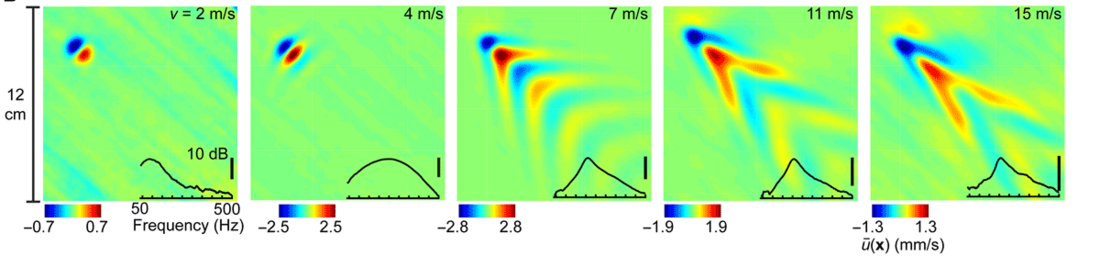
测量结果与数值模拟高度吻合,以下扫描速度为 v = 4 m/s和 7 m/s的动态演示对此进行了展示。
接下来,维塞尔团队对人体手部进行了测量(图 3)。在这些实验中,超声扫描路径沿着手掌面的轴线,从手腕延伸到食指指尖。由于横向调制运动方向(形成锯齿状路径)能引发更强的感觉,因此线性路径会进行横向调制。最低和最高扫描速度(VL = 1 m/s和 11m/s)分别处于亚音速和超音速范围。
与理论以及数值模拟、组织模型实验结果一致,低扫描速度(VL = 1 m/s)激发的剪切波模式从瞬时焦点位置向外扩展。较高扫描速度(VL > 4 m/s)产生的波型会形成尾流,尾流落后于运动轨迹 10 cm或更长。这些波型的长度比近似焦点宽度(0.6 cm)大一个数量级以上。
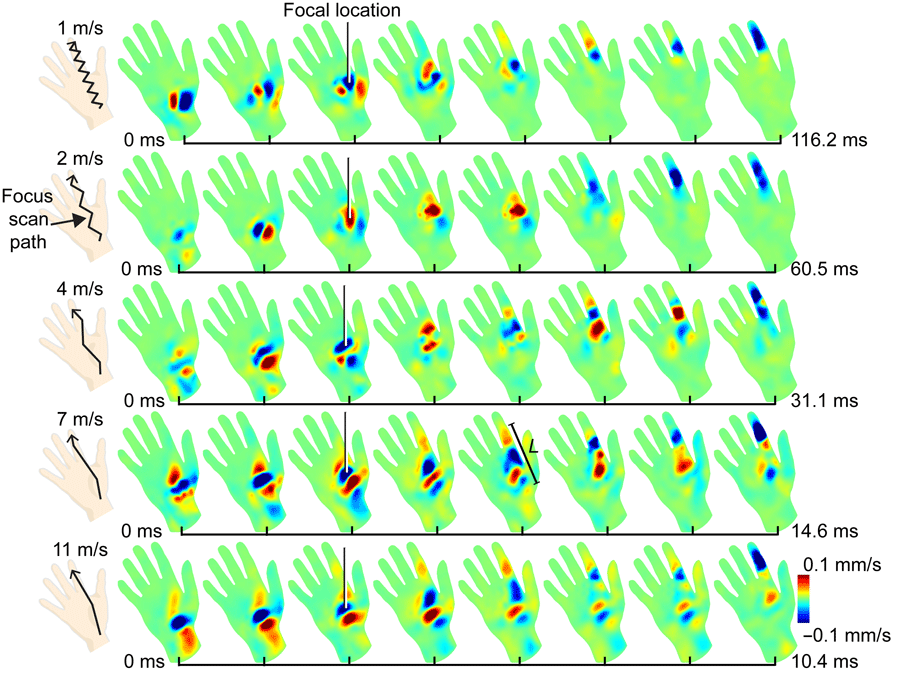
这些现象在人类触觉感知中有所体现,通过对比体内振动测量测试数据(图 4A,蓝色)和触觉运动感知实验获得的行为数据(图 4A,红色)就能看出。在每次实验中,参与者感受从手腕扫描到食指指尖或反向扫描的聚焦超声刺激,并报告扫描运动方向。较低扫描速度下的感知准确率更高(P < 0.0001),在最高的三个扫描速度(VL = 4 m/s、7 m/s和 11 m/s)下,感知准确率降至随机水平,这几个速度产生的尾流最长。综合分析感知实验和振动测量实验数据后发现,感知准确率会随着尾流长度增加而单调下降(图 4B)。
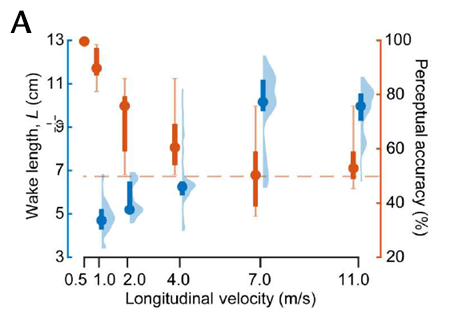
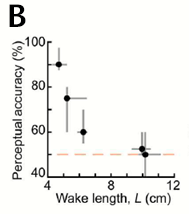
该团队发现的这一此前未知、作为触觉全息术基础的激波现象,为未来打造全息触觉显示器迈出了重要一步,有望让用户在元宇宙中实现更逼真、沉浸的交互体验。
Images courtesy of the authors unless otherwise specified. Cover image: jamesteohart/shutterstock.com