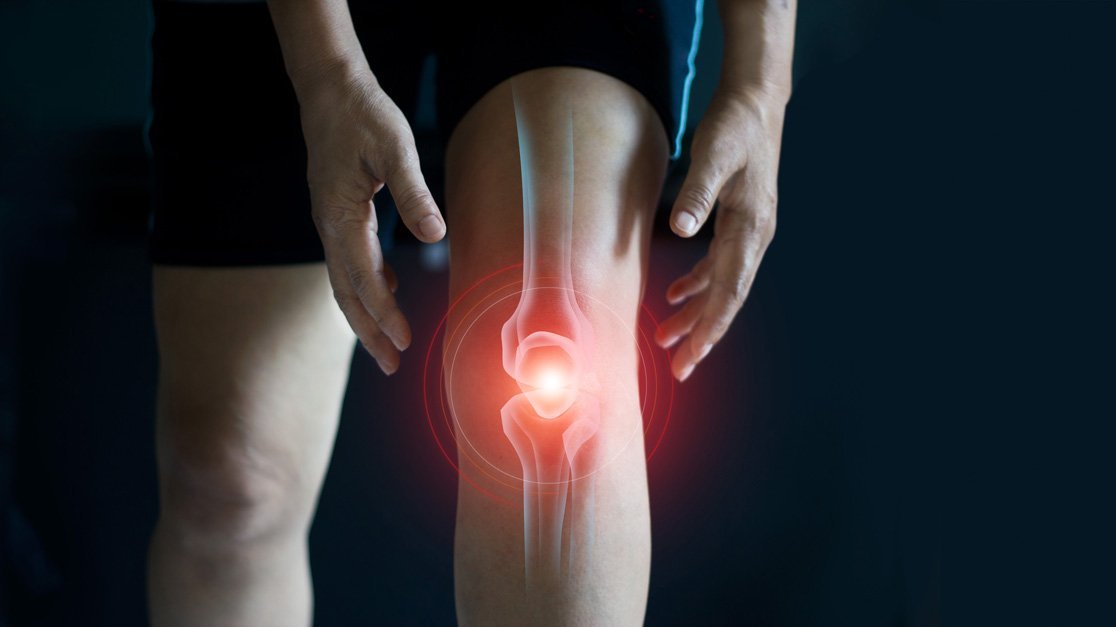
Osteoarthritis, a degenerative disease in the joint, is a condition that is afflicting approximately 14% of adults. Understanding the dynamics of the joint cartilage is crucial for research and for finding effective treatment methods. Laser Doppler vibrometry has been finding adoption in many biological sciences over the years, for example in hearing research or in the study of the skin response for haptics research. Upon hearing about vibrometry during her time at UC Irvine's UCI Samueli School of Engineering Dr. Gabriela Espinosa, now at Concordia University Irvine, was inspired to evaluate this measurement technology for the dynamic characterization of cartilage in the human knee.
With the support of distinguished professor Dr. Kyriacos Athanasiou and in collaboration with Polytec, Dr. Espinosa was successful in developing a technique to characterize the mechanical properties of cartilage. In her recent publication, she shows the agreement with conventional methods and highlights the benefits of using vibrometry instead. Due to its non-contact approach, vibrometry is, unlike conventional techniques, non-destructive and thus helps reduce waste and keep costs down.
Also, the vibrometry based method Dr. Espinosa developed, resulted in significantly reduced test times enhancing the speed in research efforts going forward. Dr. Espinosa plans to utilize this method to evaluate tissue-engineered cartilage’s similarity to native knee cartilage.
In her article, Dr. Espinosa shows how the dynamic modulus G* can be extracted from the measured resonance frequencies of a disk-shaped test sample when looking at only the 1st and 2nd order modes. Using phase/time delay measurements, the viscoelastic properties (loss modulus G” and storage modulus G’) can be extracted. Thus, with a single tool, vibrometry greatly facilitates meeting multiple regulatory recommendations for mechanical characterization of cartilage replacements.
Cartilage is a dynamically loaded tissue. Typical activities, such as walking, can generate large stresses between 1 and 6 MPa but may even peak up to 12 MPa. These stresses are applied cyclically at frequencies ranging from 0.01 to 2 Hz, typically resulting in up to 4 million loading cycles per year. Several factors, such as cyclical mechanical fatigue, excessive strains and rapid strain rates, are often implicated with the onset of osteoarthritis, a condition afflicting approximately 14% of adults.
Given its relevance to daily activities and role in maintaining tissue homeostasis, cartilage dynamic mechanical properties are a crucial component to the field’s ongoing research endeavours and to those seeking to replace or regenerate cartilage’s function in a dynamic environment.
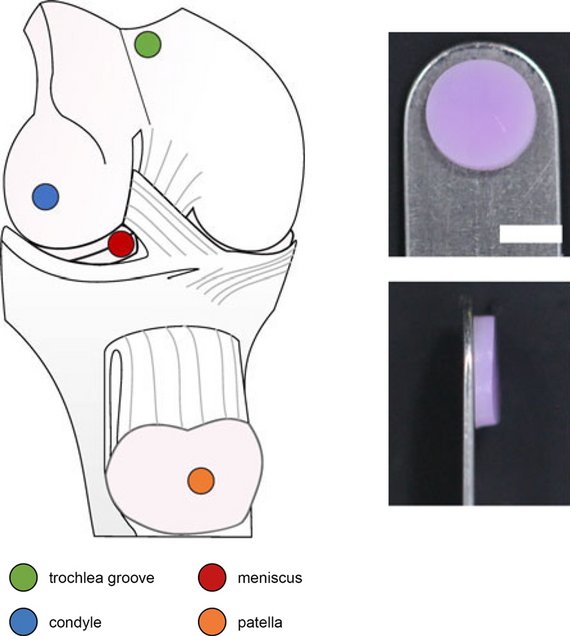
Quasi-static mechanical measurements using viscoelastic testing conditions, such as stress relaxation and creep indentation, are routinely employed to evaluate cartilage and its replacements. Dynamic mechanical testing is often performed to obtain a dynamic modulus and, occasionally, viscoelastic properties. However, most of these mechanical testing modalities are destructive, which either limits the pursuit of certain investigations or requires a large amount of testable tissue.
It is certain that a non-contact form of dynamic mechanical testing would garner much attention among cartilage researchers. A major advantage of laser Doppler vibrometry showcased in these works is the ability to perform non-contact and non-invasive measurements. Given this key characteristic, vibrometry is well poised to become a valuable measurement modality in the biological sciences.
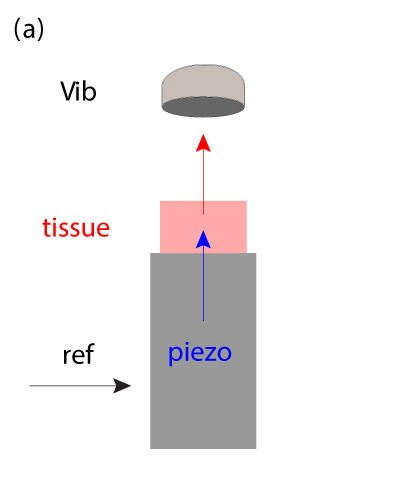
The objective of this work was to investigate the use of vibrometry as a non-contact dynamic mechanical testing modality in comparison to quasi-static mechanical and biochemical assays for articular cartilage characterization. For that purpose cartilage explants from the condyle, patella, trochlear groove and meniscus were characterized using traditional methods and vibrometry and the results were comparted.
For the vibrometry measurements a PSV-500-B Xtra Scanning Vibrometer was used. The cartilage tissue explants were placed on a piezo actuator for dynamic excitation. The function generator of the PSV-500-B Xtra Scanning Vibrometer was used to drive the piezo with the relevant signals (ref) for the respective experiments. The dynamic excitation of the tissue results in tissue sample deformation. The axial displacement of the tissue’s top surface is measured by the vibrometer (vib).
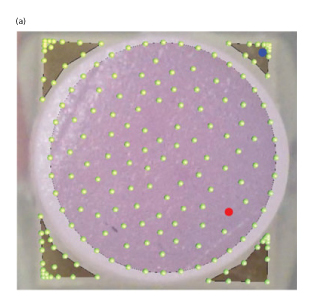
A grid (100 – 150 points) covering the tissue sample and the piezo was defined to establish the measurement points. It is important to note that measurements were taken of both the sample and the piezo.
In a first measurement, the first and second resonant frequencies were found by identifying peaks in the sample velocity data across a frequency sweep ranging from 10 to 100,000 Hz. For this analysis, data from the piezo were only used to confirm that the piezo’s resonant frequencies do not interfere with those of the sample.
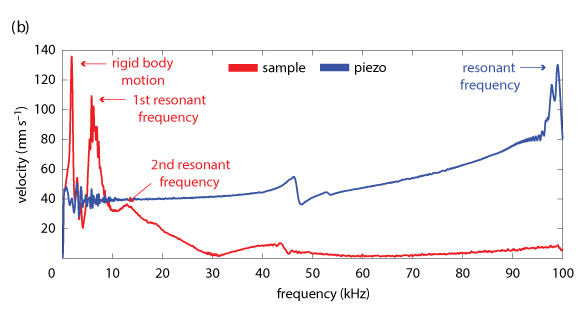
To differentiate from peaks due to rigid body motion, dynamic deflection shapes at resonance frequencies were observed to verify the presence of a bending mode.
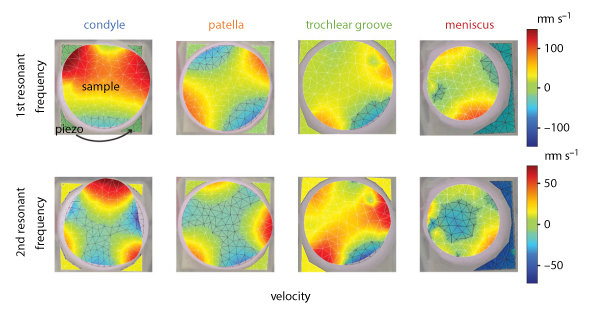
The video clip showcases animations of the first and second modes of a tissue sample as derived from the PSV Software.
The dynamic modulus, G*, of each sample was determined based on the American Society for Testing and Materials International (ASTM International) guidelines for calculating dynamic mechanical properties for disc-shaped specimens via vibration. Briefly, for each resonant frequency, a dynamic modulus was calculated. The dynamic moduli for both frequencies were averaged to give a single G* value per sample.
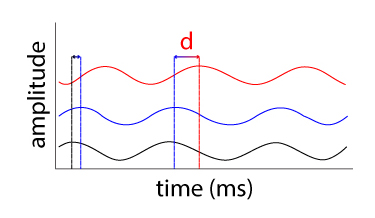
This was followed by a 500 Hz sinusoidal excitation to determine the viscoelastic properties of the tissue. A sinusoidal reference signal (black) drives the slightly shifted piezo’s response (blue). The shift is shown as the difference between the black and blue dotted lines. The tissue’s periodic deformation is shown in red, with the phase shift with respect to the piezo signal given by δ.
Using the PSV software, an inverse Fourier transform was used to convert data from the frequency domain to the time domain. In this analysis, data from both the piezo and sample were necessary
to measure the phase shift, δ, between the piezo’s and tissue’s response to the reference signal. The tangent of the phase shift is known to be the ratio of viscous to elastic contributions, also known as the loss and storage moduli, respectively. Using G* and δ, the storage modulus G’ and the loss modulus G’’ were calculated as follows:
G' = G* cos δ
G'' = G* cos δ,
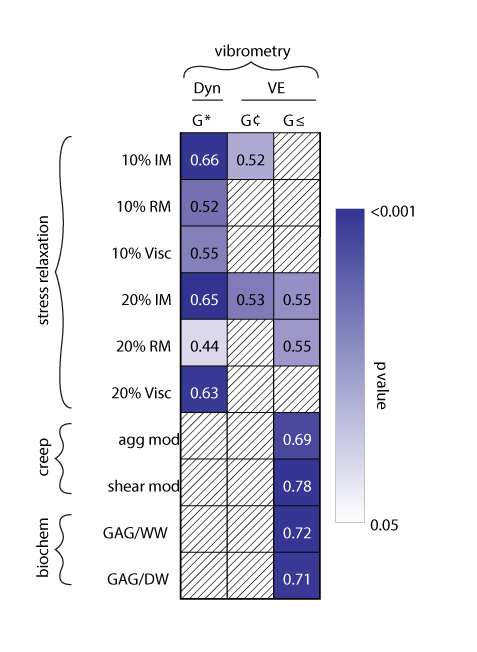
As the tissue samples were not damaged by the vibrometry measurements, they could then be used to perform the conventional destructive mechanical testing to correlate the results with those obtained from vibrometry measurements. Namely stress relaxation, creep indentation and a biochemical assay were performed.
The table summarizes the strength and significance of Spearman ρ correlations of dynamic modulus G*, the storage modulus G’ and the loss modulus G’’ derived with non-destructive vibrometry measurements and traditional destructive assays used in cartilage research.
The numbers indicate the strength of the correlation (ρ), and colour indicates the degree of significance. White boxes with diagonal lines denote that statistical significance was not achieved.
This study successfully shows the use of vibrometry in a non-contact manner to measure cartilage’s dynamic mechanical properties. The dynamic properties reported here showed several correlations with quasi-static and biochemical properties, thus providing important insights into cartilage function under cyclic loading. Vibrometer-based measurements were also precise enough to detect differences in viscoelastic properties among the cartilages of the knee.
This work not only solidifies the relationship among quasi-static, viscoelastic and dynamic properties over a short testing time but also presents a practical option for high-throughput non-destructive testing, ideal for applications in cartilage developmental biology, tissue engineering and translational medicine.
Read more in this research article https://lnkd.in/gh2ek6kB
Images courtesy: Images courtesy of the authors unless otherwise specified. Cover image: PopTika/shutterstock.com